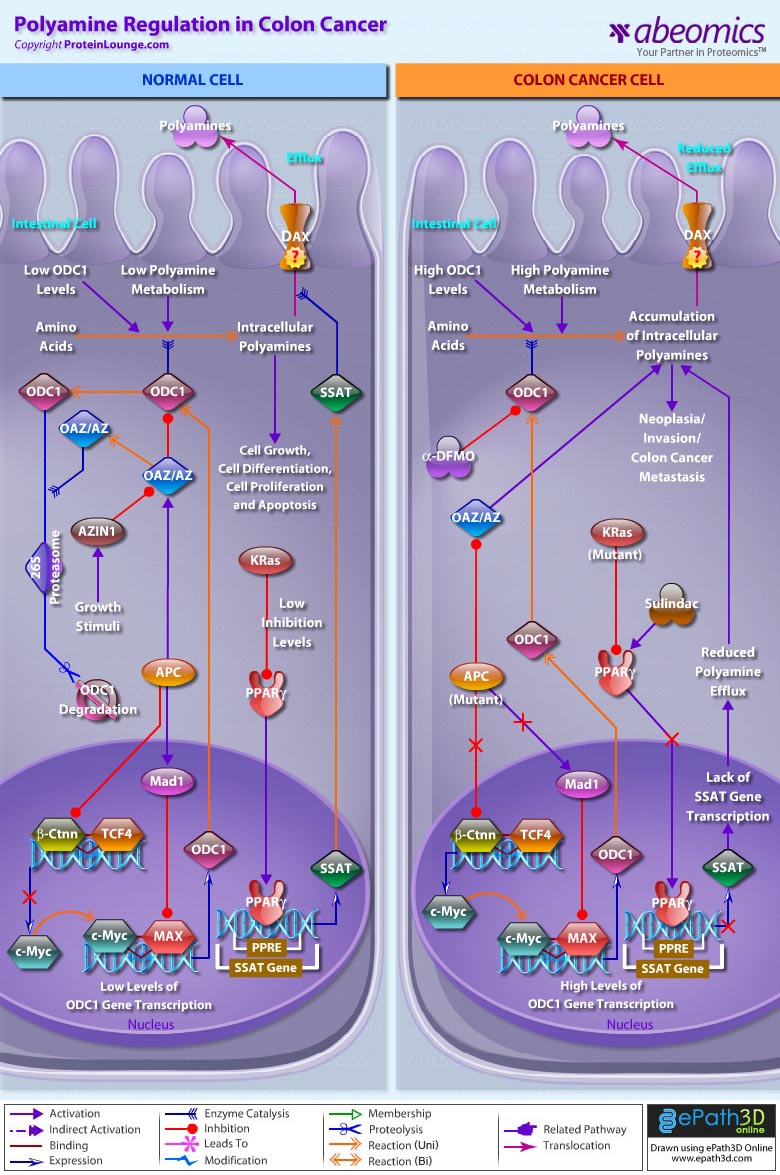
Polyamines are vital for the growth and function of normal cells. The complexity of polyamine metabolism and the multitude of compensatory mechanisms that are invoked to maintain polyamine homoeostasis argue that these amines are critical to cell survival. The regulation of polyamine content within cells occurs at several levels, including transcription and translation (Ref.1). The amino-acid derived polyamines like Putrescine, Spermidine and Spermine that are the main polyamines found in prokaryotes and eukaryotes, have long been associated with cell growth and cancer, and specific oncogenes and tumor suppressor genes regulate polyamine metabolism. Regulation of these polyamines (otherwise known as “organic cations”) is chiefly associated with cancers pertaining to the colon. They are formed by the enzymatic decarboxylation of the amino acids like Ornithine or Arginine by ODC1 (Ornithine Decarboxylase-1) the enzyme required for the first stage in polyamine synthesis. ODC1 is subject to both positive and negative feedback regulation by polyamines: high polyamine concentrations decrease, and low polyamine concentrations increase, activity (Ref.2 & 3).
In normal cells, low ODC1 and polyamines level is essential for normal cell growth and differentiation, cell proliferation and apoptosis, for which, the feedback regulation of ODC1, is a mixture of post-transcriptional regulation and the induction of a unique ODC1-specific inhibitor termed OAZ (Ornithine Decarboxylase Antizyme). OAZ binds to ODC1 and the OAZ-ODC1 complex is degraded by the 26S Proteasome. Unusually, the degradation of ODC1 by this Proteasome occurs in an ATP-dependent, but Ub (Ubiquitin)-independent, manner. ODC1 is released from OAZ by another unique protein, called AZIN1 (Antizyme Inhibitor-1), which liberates ODC1 in the presence of growth stimuli by virtue of having a higher affinity for OAZ than for ODC1. Additionally, OAZ can alter polyamine homoeostasis by down-regulating polyamine uptake independent of the effects on ODC1. It may be that OAZ binding to ODC1 causes a conformational change in ODC1, resulting in exposure of its C-terminus, so targeting it for degradation. Also expression of full-length APC (Adenomatous Polyposis Coli) results in up-regulation of OAZ and APC-dependent expression of Mad1 forms hetero-complexes with the c-Myc binding partner, MAX (Myc-Associated Factor-X). c-Myc acts as a transcriptional activator and Mad1 as the transcriptional repressor for ODC1 gene expression. Mad1 compete with c-Myc for binding to MAX, thereby decreasing the levels of c-Myc:MAX complexes. An increased ratio of Mad1:MAX to c-Myc:MAX hetero-complexes lead to greater binding at e-box domains by Mad1:MAX complexes. So the expression of c-Myc is down-regulated during differentiation, whereas Mad1 expression is up-regulated and the reciprocal effect results in cell growth by maintaing low levels of ODC1 and polyamines. APC further inhibits Ctnn-Beta (Catenin-Beta)/TCF4 (Transcription Factor-4) association to down-regulate ODC1 gene expression (Ref.1 & 4). In addition, SSAT (Spermidine/SpermineN1-Acetyltransferase) gene transcription is negatively regulated by the KRas (Kirsten-Ras) oncogene through a mechanism involving PPAR-Gamma (Peroxisome Proliferative Activated Receptor-Gamma), a putative tumor suppressor. PPAR-Gamma positively regulates the transcription of SSAT through PPRE (PPAR Response Element) in the SSAT promoter. KRas suppresses SSAT transcription by inhibiting PPAR-Gamma expression and subsequent binding to the SSAT promoter. This maintains polyamine homeostasis and regulates polyamine efflux through DAX (Diamine Exporter). The mechanisms of this polyamine export, or efflux from mammalian cell types, are not yet defined (Ref.3).
In contrast, when wild-type APC is lost in intestinal epithelia, during development of colon cancer, decreased OAZ activity contributes to increased ODC levels. Defects in the APC gene may occur in at least 90% of colon adenomas and cancers and are responsible for both inherited and sporadic forms. However, the mechanisms by which APC mutations promote tumorigenesis are poorly understood (Ref.4). It is known that tumor cells with truncated APC exhibit high levels of Ctnn-Beta because it cannot be targeted to the 26S Proteasome for degradation. This leads to Ctnn-Beta/TCF4 association and translocation to the nucleus where transcription of target gene, c-Myc is activated, leading to high levels of ODC and intracellular polyamine accumulation. Similarly, KRas which is commonly mutated and aberrantly activated in human colon cancer and other gastrointestinal cancers strongly inhibits PPAR-Gamma expression and subsequent binding to the SSAT promoter. This results in loss of polyamine efflux and facilitates neoplasia/invasion by cancer cells. So, polyamine synthesis and catabolism are both regulated by signaling pathways that are influenced by oncogenes and tumour-suppressor genes. Aberrant APC and KRas expression are essential for neoplasia indicate how polyamine levels become increased in colon and other gastrointestinal cancers (Ref.5 & 6).
Polyamines continue to be molecules that hold fascination for biologists, chemists, molecular biologists and clinical researchers. Polyamine levels can increase because of synthesis and/or uptake, and their metabolism is highly regulated by a series of catabolic enzymes in mammals. Consequently, it should not be surprising that attaining the goal of reducing high polyamine levels in tissues during cancer development might require targeting two or more of these processes. The concept of combination therapy for chemoprevention is important, as combinations of agents are generally more effective than single agents. Although anticancer agents such as Alpha-DFMO (Alpha-Difluoromethylornithine) (inhibit ODC1) and Sulindac (activates PPAR-Gamma) function, are effective in treating colon cancer yet the future may witness adoption of new functional genomics approaches like RNAi technology that provides the opportunity to pursue an exciting new therapeutic approach to treat colon cancers (Ref.7 & 8).
References:
1.
A perspective of polyamine metabolism.
Wallace HM, Fraser AV, Hughes A.
Biochem. J. 2003 Nov 15;376(Pt 1):1-14.
2.
Polyamines and cancer: old molecules, new understanding.
Gerner EW, Meyskens FL Jr.
Nat. Rev. Cancer. 2004 Oct;4(10):781-92.
3.
Signaling pathways in intestinal development and cancer.
Sancho E, Batlle E, Clevers H.
Annu. Rev. Cell Dev. Biol. 2004;20:695-723.
4.
APC-dependent regulation of ornithine decarboxylase in human colon tumor cells.
Fultz KE, Gerner EW.
Mol. Carcinog. 2002 May;34(1):10-8.
5.
Polyamines as modifiers of genetic risk factors in human intestinal cancers.
Babbar N, Gerner EW.
Biochem. Soc. Trans. 2003 Apr;31(2):388-92.
6.
Polyamines and colon cancer.
Milovic V, Turchanowa L.
Biochem. Soc. Trans. 2003 Apr;31(2):381-3.
7.
RNAi technology: a revolutionary tool for the colorectal cancer therapeutics.
Lv W, Zhang C, Hao J.
World J. Gastroenterol. 2006 Aug 7;12(29):4636-9.
8.
Cyclooxygenase-independent induction of apoptosis by sulindac sulfone is mediated by polyamines in colon cancer.
Babbar N, Ignatenko NA, Casero RA Jr, Gerner EW.
J. Biol. Chem. 2003 Nov 28;278(48):47762-75