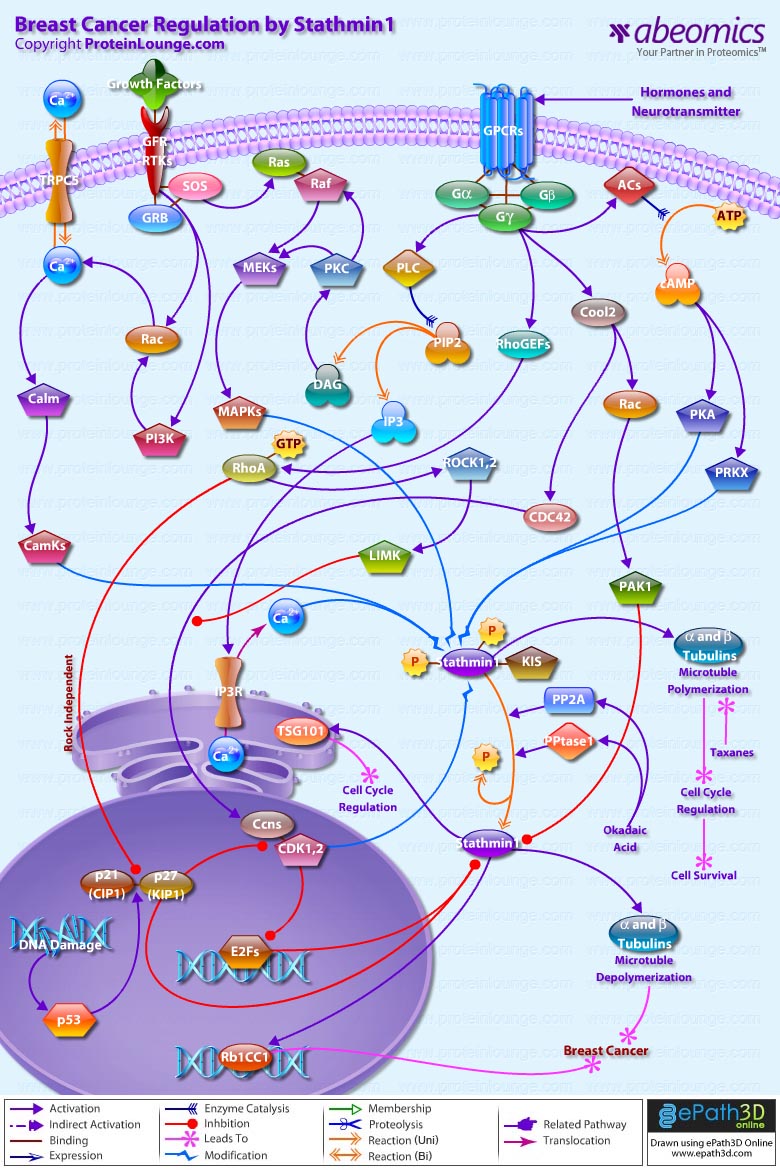
Stmn1 (Stathmin-1) also referred to as Op18 (Oncoprotein-18) is a major regulator of microtubule dynamics. It is an evolutionarily well conserved 17 kDa cytoplasmic phosphoprotein that is highly expressed in a wide variety of cancers and its high abundance seems to be necessary for the maintenance of the transformed phenotypes. Breast cancers exhibit high levels of Stmn1 and may be resistant to anti-microtubule agents. Stmn1 destabilizes microtubule polymers of Alpha and Beta-Tubulin subunits, by promoting catastrophes that ultimately results in deregulation of cell cycle, hampering cell survival (Ref.1). One of the key properties of microtubules is that of ‘dynamic instability’. Dynamic instability comprises the continuous switching between catastrophes (depolymerization or shrinkage phase) and rescues (polymerization or growing phase) of individual microtubules. Stmn1 expression is ubiquitous and is phosphorylated on up to four sites (Ser16 (Serine-16), Ser25, Ser38 and Ser63) in response to many regulatory signals within cells, in order to maintain equilibrium during microtubule polymerization. Its molecular characterization indicates a functional organization including an N-terminal regulatory domain that bears the phosphorylation sites, linked to a putative Alpha-helical binding domain predicted to participate in coiled-coil, protein-protein interactions (Ref.2).
In addtion to the protein kinases that phosphorylate Stmn1 such as CamKs (Calcium/Calmodulin-Dependent Protein Kinases), MAPKs (Mitogen-Activated Protein Kinases), CDKs (Cyclin-Dependent Kinases), PKA (cAMP dependent Protein Kinase-A), PRKX (Protein Kinase-X-Linked); a few other proteins are known to interact with Stmn1 in vivo. One of them is a putative Serine/Threonine kinase, KIS (Kinase Interacting Stathmin), which binds to Stmn1 and is regulated by it or, more likely, is a part of the kinases controlling phosphorylation state of Stmn1. Two other proteins, Rb1CC1 (Rb1-Inducible Coiled-Coil-1) and TSG101 (Tumor Susceptibility Gene-101), form Alpha-helices participating in coiled-coil interacting structures to regulate cell cycle (Ref.3 & 4). The upstream factors that activate the kinases modulating Stmn1 function, includes activation by hormones and neurotransmitters through GPCRs (G-Protein Coupled Receptors); activation by Growth Factors through Growth Factor Receptors and RTKs (Receptor Tyrosine Kinases); and Ca2+ (Calcium) influx through channel proteins like TRPC5 (Transient Receptor Potential Cation Channel Subfamily-C Member-5) and IP3Rs (IP3 Receptors). Hormones and neurotransmitters stimulate the G-proteins (Alpha, Beta and Gamma-subunits) that activate downstream effectors like ACs (Adenylate Cyclases), Cool2, RhoGEFs (Rho Guanine Nucleotide Exchange Factors) and PLC (Phospholipase-C) in order to intensify phoshorylation of Stmn1. The tmACs (Trans-membrane Adenylate Cyclase) enzymes are responsible for the accumulation of cAMP (Cyclic Adenosine 3',5'-monophosphate) from ATP (Adenosine Triphosphate). cAMP directly regulates the activities of PKA (cAMP-dependent Protein Kinase-A) and PRKX (Protein Kinase-X-Linked) leading to phosphorylation of Stmn1 at Ser16 and Ser63. G-proteins activate PLC (Phospholipase-C) to cleave PIP2 (Phosphatidylinositol 4,5-bisphosphate) generating DAG (Diacylglycerol) and IP3 (Inositol 1,4,5-trisphosphate). DAG activates PKC (Protein Kinase-C), whereas, IP3 modulates Ca2+ release through IP3R (IP3 Receptor). Activation of PKC acts in concert with Growth Factor Receptor/RTK associated proteins like GRB (Growth Factor Receptor-Bound Proteins) and SOS (Son of Sevenless) to phosphorylate Stmn1 at Serine position 25 through a signaling cascade that includes, Ras, Raf, MEKs (MAPK/ERK Kinases), and MAPKs (Mitogen-Activated Protein Kinases). GRB and SOS also activate Rac and PI3K (Phosphatidylinositde-3-Kinase) to modulate release of Ca2+ ions through TRPC5 (Transient Receptor Potential Cation Channel Subfamily-C Member-5). This further activates Calm (Calmodulin) and CamKs (Calcium/Calmodulin-Dependent Protein Kinases), which along with Ca2+ ions act synergistically with PKA and PRKX to phosphorylate Stmn1 at Ser16 (Ref.5 & 6).
Further G-protein signaling couples Rac and CDC42 (Cell Division Cycle-42) activation, with RhoA activation, to phosphorylate and inactivate Stmn1. G-proteins stimulate Guanine Nucleotide Exchange Factors like RhoGEFs and Cool2 to activate RhoA and Rac-CDC42, respectively. Rac and CDC42 relay signals to PAK1 (p21/CDC42/Rac1-Activated Kinase-1) and Ccns (Cyclins) that in turn inhibit anti-microtubule action of Stmn1 through phosphorylations (Ref.7). Rac-CDC42 and RhoA function in parallel pathways to stimulate Ccn expression. The targets of RhoA include Rho-Kinase/ROCK (Rho-Associated Coiled-Coil-Containing Protein Kinase) and LIMK (LIM Kinase). Activity of Rho-ROCK-LIMK signaling promotes Ccn expression and suppresses Rac-CDC42 activation of Ccn. So, reducing Rho-ROCK-LIMK signaling would stimulate Rac-CDC42 dependent Ccn expression (Ref.3).
RhoA acting independently of ROCK, also modulates the cell cycle by regulating expression of p21(CIP1) (Cyclin Dependent Kinase Inhibitor-p21) and p27(KIP1) (Cyclin Dependent Kinase Inhibitor-p27) and this reflects on Stmn1 phosphorylation and Tubulin dynamics. p27(KIP1) binds to RhoA and prevents the interaction of RhoA with its activators. The transcriptional regulation of p21(CIP1) and p27(KIP1) is controlled by p53 and this signifies the up-regulation of Stmn1 in human breast cancers, under conditions like mutations or DNA damage (Ref.8). p27(KIP1) has the potential for modulating Stmn1 expression and/or function through at least four different mechanisms: (i) p27(KIP1) might directly interact with Stmn1 and prevent its microtubule destabilizing activity; (ii) p27(KIP1) may activate Rac, which can promote the phosphorylation of Stmn1 and inhibit its microtubule destabilizing activity; (iii) the inhibitory effect of p27(KIP1) on CDKs might result in either E2F transcription factor mediated down regulation of Stmn1 expression or increased Stmn1 activity by means of CDK inactivation; (iv) p27(KIP1) and Stmn1 might interact through KIS that phosphorylates p27(KIP1) and induces its translocation to the cytoplasm where Stmn1 is localized (Ref.9 & 10). However dephosphorylation of Stmn1 by an Okadaic Acid-sensitive PPtase1 (Protein Phosphatase-1) and PP2A (Protein Phosphatase-2A) is crucial for the ability of cells to exit mitosis. A similar effect is also shown by Taxanes, group of chemotherapy drugs that includes Taxol and Taxotere, specifically used to kill cancer cells of mammary gland by stopping their growth (Ref.11).
Stmn1 is a major microtubule-destabilizing protein that promotes microtubule depolymerization by two distinct mechanisms. The first is a catastrophe-promoting microtubule depolymerization activity that is necessary for the regulation of the mitotic spindle. The second is a Tubulin-sequestering activity that inhibits microtubule polymerization and is necessary for the regulation of microtubule dynamics during interphase (Ref.12). Both activities are regulated by phosphorylation, which inactivates Stmn1 and prevents its binding to Tubulin and interferes with the sensitivity of breast cancer cells to anti-microtubule drugs (Ref.13).
References:
1.The oncoprotein 18/stathmin family of microtubule destabilizers.
Cassimeris L.
Curr. Opin. Cell Biol. 2002 Feb;14(1):18-24.
2.The effect of stathmin phosphorylation on microtubule assembly depends on tubulin critical concentration.
Amayed P, Pantaloni D, Carlier MF.
J. Biol. Chem. 2002 Jun 21;277(25):22718-24.
3.Regulation of the cytoskeleton: an oncogenic function for CDK inhibitors?
Besson A, Assoian RK, Roberts JM.
Nat. Rev. Cancer. 2004 Dec;4(12):948-55.
4.Overexpression of tumor susceptibility gene TSG101 in human papillary thyroid carcinomas.
Liu RT, Huang CC, You HL, Chou FF, Hu CC, Chao FP, Chen CM, Cheng JT.
Oncogene. 2002 Jul 18;21(31):4830-7.
5.Identification of Op18/stathmin as a potential target of ASK1-p38 MAP kinase cascade.
Mizumura K, Takeda K, Hashimoto S, Horie T, Ichijo H.
J. Cell Physiol. 2005 Aug 18.
6.The PI 3-kinase-Rac-p38 MAP kinase pathway is involved in the formation of signet-ring cell carcinoma.
Xu Q, Karouji Y, Kobayashi M, Ihara S, Konishi H, Fukui Y.
Oncogene. 2003 Aug 28;22(36):5537-44.
7.Conserved microtubule-actin interactions in cell movement and morphogenesis.
Rodriguez OC, Schaefer AW, Mandato CA, Forscher P, Bement WM, Waterman-Storer CM.
Nat. Cell Biol. 2003 Jul;5(7):599-609.
8.Overexpression of stathmin in breast carcinomas points out to highly proliferative tumours.
Curmi PA, Nogues C, Lachkar S, Carelle N, Gonthier MP, Sobel A, Lidereau R, Bieche I.
Br. J. Cancer. 2000 Jan;82(1):142-50.
9.p27(Kip1) and stathmin share the stage for the first time.
Iancu-Rubin C, Atweh GF.
Trends Cell Biol. 2005 Jul;15(7):346-8.
10.E2F mediates sustained G2 arrest and down-regulation of Stathmin and AIM-1 expression in response to genotoxic stress.
Polager S, Ginsberg D.
J. Biol. Chem. 2003 Jan 17;278(3):1443-9.
11.Stathmin inhibition enhances okadaic acid-induced mitotic arrest: a potential role for stathmin in mitotic exit.
Mistry SJ, Atweh GF.
J. Biol. Chem. 2001 Aug 17;276(33):31209-15.
12.The role of stathmin in the regulation of the cell cycle.
Rubin CI, Atweh GF.
J. Cell Biochem. 2004 Oct 1;93(2):242-50.
13.Effect of stathmin on the sensitivity to antimicrotubule drugs in human breast cancer.
Alli E, Bash-Babula J, Yang JM, Hait WN.
Cancer Res. 2002 Dec 1;62(23):6864-9.